The Importance of Adequate Hormone Function in Female Athletes
- Issy Coombes
- Nov 19, 2021
- 8 min read
The menstrual cycle is an important biological rhythm that involves cyclical and predictable fluctuations in the endogenous sex hormones oestrogen and progesterone. Traditionally a textbook cycle is defined as 28 days (figure 1), however, this length can vary from 21-35 days and is unique for every individual. The menstrual cycle is characterised by two major phases, the follicular phase (blue) and the luteal phase (pink). These phases are split by ovulation (release of an egg) which occurs approximately halfway through the cycle.
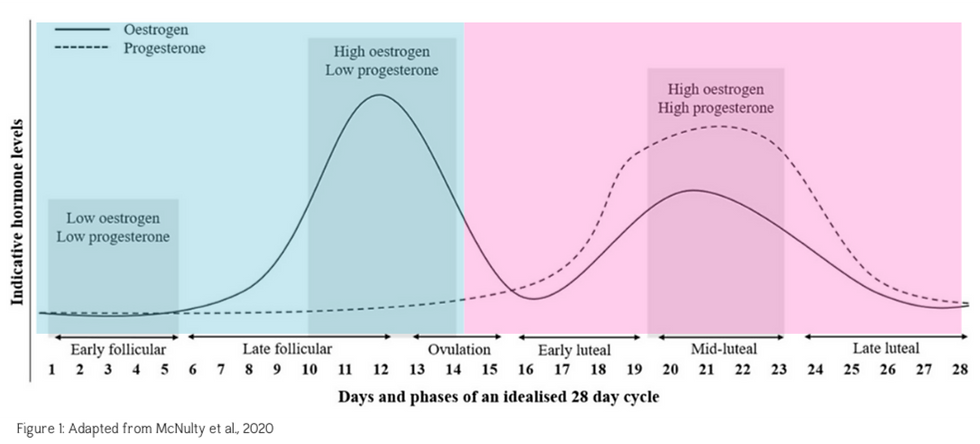
The process of menstruation is controlled by neuroendocrine glands (the reproductive axis) and its appropriate function is linked to signals associated with pubertal development, somatic growth, body composition, stress responses, appetite, energy expenditure and insulin action. This regulation is reliant on negative and positive feedback mechanisms, as well as inputs from the central nervous system and other systems at various levels within the axis (figure 2). Signalling starts in the hypothalamus, the brains control centre, which secretes a hormone called gonadotropin releasing hormone (GnRH). GnRH subsequently acts on the pituitary, the master endocrine gland, which then synthesises and secretes luteinising hormone (LH) and follicle stimulating hormone (FSH). The general circulation delivers LH to the ovaries and this stimulates the synthesis and release of oestrogen and progesterone. Oestrogen and progesterone reversibly suppress (feedback on) the hypothalamus, thereby causing intermittent secretion of GnRH, LH and FSH. Essentially, GnRH pulsation must occur at an optimal frequency if the whole axis is to function normally and you are to experience a regular menstrual cycle.
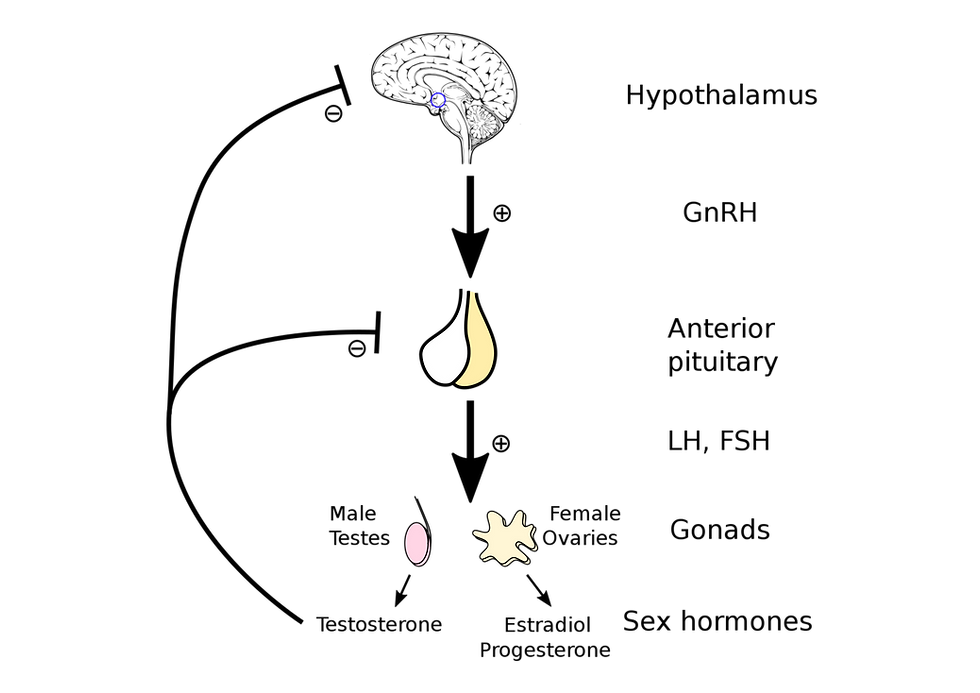
Figure 2: Hypothalamic Pituitary Ovarian Axis
Research in female endurance athletes has highlighted that a large percentage of individuals present with irregular (oligomenorrhea) or completely absent (amenorrhea) menstrual cycles. Even though menstruation is a fundamental aspect of female physiology, several factors can influence GnRH secretion from the hypothalamus and therefore cause disruption to the entire HPO axis. Amenorrheic athletes have consistently low levels of oestrogen and progesterone which indicates a complete absence of ovulation, and fertility (8). In addition, even eumenorrheic athletes with a regular menstrual cycle can exhibit extended follicular phases and condensed luteal phases with blunted progesterone concentrations compared to eumenorrheic sedentary women. These findings have been seen in eumenorrheic females recreational runners completing a mere 20 kilometres per week. Unfortunately, these less severe disruptions (follicular suppression, luteal suppression and anovulation) may not produce any menstrual symptoms therefore, females can be completely unaware of their condition. This was demonstrated in a study by De Souza et al. (1998) where repeated blood work found that 79% of eumenorrheic female runners had luteal phase suppression or were anovulatory in at least one month out of three.
The main cause of this menstrual dysfunction in both amenorrheic and eumenorrheic athletes is a disruption in LH pulsation. Further experiments administrating external forms of GnRH have demonstrated that this disruption to LH pulsality occurs at the level of the hypothalamus. Therefore, throughout the remainder of this article we will look to understand the main neuroendocrine mechanisms that enable adequate functioning of the hypothalamus and consequently GnRH pulsation for a regular and effective menstrual cycle.
Originally, the chronic elevation of cortisol, the body’s stress hormone, seen in amenorrheic athletes caused scientists to theorise that the stress of exercise impacted female reproductive function. However, upon further examination, studies found that athletes with menstrual irregularities also displayed low levels of blood sugar, insulin growth factor, leptin, thyroxin and had reduced resting metabolic rates. Additionally, these athletes exhibited elevated levels of growth hormone alongside their high cortisol. These biochemical markers are not signs of exercise stress but rather a chronic deficiency in energy.
Energy deficiency can be defined as, “an inadequacy of energy to support the range of body functions involved in optimal health and performance” and is the underlying cause that affects the functioning of numerous other physiological systems. Energy intake should exceed exercise energy expenditure so that energy availability is sufficient to support training adaptations, functioning of physiological systems and ultimately optimal athletic performance. Energy availability can be estimated by calculating energy intake minus exercise energy expenditure relative to fat free mass (muscle tissue). In a controlled laboratory setting with non-athletic females, EA<30kcal/kg FFM disrupted leutinising hormone (LH) pulsatility in comparison to females achieving an EA~45kcal/kg FFM. The degree of LH disruption was significantly increased with the severity of EA (further reductions in kcal per kg FFM) however, this variable along with the impact of duration and frequency of LEA and the extent of disruption of the females physiological functioning is still an active area of investigation.
Whilst you may be thinking, “I don’t have chronic energy deficiency because I’m not losing weight”. Well, you could be incorrect. A stable body weight is not necessarily proof of adequate energy availability, since alterations to behaviour and resting metabolic rate can counteract the influence of energy deficiency on body mass. As a survival mechanism, the body will always prioritise energy for locomotion and therefore, in the absence of adequate energy this limits the fuel available for other physiological systems including cellular maintenance, thermoregulation, immunity, locomotion, growth and reproduction. Furthermore, generating accurate calculations of energy availability present an array of difficulties and it is expected that this will be accompanied by errors of validity and reliability with mean estimates varying by up to 600 calories per day.
On average your brain needs 500 calories per day and since fatty acids cannot cross the blood-brain barrier, this must come in the form of glucose from carbohydrate. Failure to provide sufficient fuel to meet the brains energy requirements will cause an alteration in brain function that disrupts the hypothalamus and therefore, production of GnRH. The brain has no fuel storage capacity yet due to its size and function it also uses more energy that can be stored in the liver as glycogen. In addition, because skeletal muscle lacks the enzyme to return glucose derived from muscle glycogen to the bloodstream, muscle glycogen stores are also not available to the brain. However, skeletal muscle also have access to liver glycogen stores and is essentially in competition with the brain for endogenous stores of carbohydrate when in an energy deficient state.
So by now you're probably thinking, "how can I ensure that I'm not in an energy deficient state?"Based on the current literature, this involves an assessment of holistic interventions that address energy availability in addition to other nutritional (within day energy balance, carbohydrate availability, fibre and calcium intake) and physical (psychological and mechanical) factors.
Within day energy balance addresses the frequency of periods of energy deficit as a result of fluctuating energy availability over a 24-hour period. This was demonstrated by Fahrenholtz et al., (2018) in their examination of WDEB among a group of elite female endurance athletes with similar 24-hour EB and EA that were separated into eumenorrheic (n=10) and menstrual dysfunction (MD) (n=15). It was reported that whilst all athletes spent a large proportion of the day in an energy deficit regardless of menstrual status, MD athletes spent 24% more time in EB<-300 kcal and 9% more time in EB<0 suggesting a more intense and frequent state of energy deficit in MD athletes.
Athletes may also benefit from increasing the proportion of carbohydrate intake relative to other macronutrients. Carbohydrate periodisation has been shown to affect bone metabolism in a study of thirty world-class race walkers (5 female, 25 male) during their build up to the 2016 Olympic Games. Additionally, carbohydrate consumption influences leptin levels which has a significant role on an individual’s nutrient intake and energy metabolism that may influence optimal functioning of the HPO axis.
Consumption of a high fibre diet can make it difficult for female athletes to meet energy requirements and therefore, increases the risk of insufficient energy intake. In one study athletes in low energy availability had a 23% higher total (g/day) and a 32% higher relative fibre intake (g/1000 kcal) compared with the eummenorheic group and concomitantly 8% lower EI, 6% higher EEE and 10% lower energy availability as a result. Consequentially, these athletes in low energy consumed a diet that was 16% lower in energy density than participants in the study with optimal energy availability.
To summarise, research consistently shows that female athletes fail to match their energy intake with high exercise energy expenditure or increasing exercise energy expenditure that can occur throughout a training and competitive season. Energy intake, nutrient timing, consumption of carbohydrate and reduced fibre ingestion have all been shown to be important for athletes to maintain adequate energy availability and normal hormonal function for optimal health and wellbeing for performance.
To see how hormonal fluctuations affect how you feel and perform thoughout your menstrual, keep a daily record. With the help of iPhone apps such as WILD AI and Fitr Woman, this is now easier than ever! If you aren’t already tracking your cycle then be sure to download one of these apps in the links below so you can take a more individual approach to how you alter training around your menstrual cycle.
If you want to get a better understanding of how hormones affect you health and wellbeing for performance then be sure to sign up for the pre-sale of my NEW course "The Essential Activities That Optimise Health and Wellbeing for Performance in Female Athletes"
References:
Benardot, D. (2013). Energy thermodynamics revisited: Energy intake strategies for optimizing athlete body composition and performance. Pensar En Movimiento: Revista de Ciencias Del Ejercicio y La Salud, 11(2), 1–14.
Burke, L. M., Lundy, B., Fahrenholtz, I. L., & Melin, A. K. (2018). Pitfalls of conducting and interpreting estimates of energy availability in free-living athletes. International Journal of Sport Nutrition and Exercise Metabolism, 28(4), 350–363.
Bursztein, S., Elwyn, D.H., Askanazi, J. & Kinney, J.M. (1989) Energy Metabolism, Indirect Calorimetry, and Nutrition. Williams & Wilkins, Baltimore, MD: 146.
De Souza, M.J., Miller, B.E., Loucks, A.B. et al. (1998). High frequency of luteal phase deficiency and anovulation in recreational women runners: blunted elevation in follicle-stimulating hormone observed during luteal follicular transition. Journal of Clinical Endocrinology and Metabolism 83(12), 4220–4232.
Fahrenholtz, I. L., Sjödin, A., Benardot, D., Tornberg, B., Skouby, S., Faber, J., : : : Melin, A. K. (2018). Within-day energy deficiency and reproductive function in female endurance athletes. Scandinavian Journal of Medicine & Science in Sports, 28(3), 1139–1146.
Heikura, I. A., Stellingwerff, T., & Areta, J. L. (2021): Low Energy Availability in Female Athletes: From the Lab to the Field. European Journal of Sport Science, DOI: 10.1080/17461391.2021.1915391
Loucks, A.B., Mortola, J.F., Girton, L. & Yen, S.S.C. (1989) Alterations in the hypothalamic–pituitary–ovarian and the hypothalamic–pituitary–adrenal axes in athletic women. Journal of Clinical Endocrinology and Metabolism 68(2), 402–411.
Loucks, A.B. & Thuma, J.R. (2003) Luteinizing hormone pulsatility is disrupted at a threshold of energy availability in regularly menstruating women. Journal of Clinical Endocrinology and Metabolism 88(1), 297–311.
Leibel, R.L., Rosenbaum, M. & Hirsch, J. (1995) Changes in energy expenditure resulting from altered body weight. New England Journal of Medicine 332(10), 621–628.
Melin, A., Tornberg, Å., Skouby, S., Møller, S. S., Faber, J., SundgotBorgen, J., & Sjödin, A. (2016). Low-energy density and high fibre intake are dietary concerns in female endurance athletes. Scandinavian Journal of Medicine & Science in Sports, 26(9), 1060–1071.
Mountjoy, M., Sundgot-Borgen, J., Burke, L., Carter, S., Constantini, N., Lebrun, C., : : : Ljungqvist, A. (2014). The IOC consensus statement: Beyond the female athlete triad-relative energy deficiency in sport (RED-S). British Journal of Sports Medicine, 48(7), 491–497.
Torstveit, M. K., Fahrenholtz, I. L., Stenqvist, T. B., Sylta, O., & Melin, A. (2018). Within-day energy deficiency and metabolic perturbation in male endurance athletes. International Journal of Sport Nutrition and Exercise Metabolism, 28(4), 419–427.
Veldhuis, J.D., Evans, W.S., Demers, L.M. et al. (1985) Altered neuroendocrine regulation of gonadotropin secretion in women distance runners. Journal of Clinical Endocrinology and Metabolism 61(3), 557–563.
Comments